The Unruh Effect and the Jump to Hyperspace. Ready to board the Millennium Falcon?
The Unruh Effect is one of the most interesting and controversial it is one of the most interesting and controversial results of quantum field theory in a flat spacetime.
When a scientific theory makes a prediction, it has to pass an experimental test in order to be considered valid. In other words, measurements have to be made. So let us imagine that we set up a theory and proceed to measure to validate it. Barring errors in the measurement process, a positive result strengthens the theory. A negative result forces the theoretical physicist to explain the discrepancy between the results obtained and those he hoped to obtain instead. This gives rise to alternative theories that are useful for explaining what does not work in the first formulation. Let’s think of it as an offshoot of daughter theories: They start from the mother theory and evolve, trying to solve what doesn't work. The main problem with the Unruh Effect is that it is difficult to measure. How do we know if it is a valid theory? And how do we develop sub-theories?
The Unruh Effect
But what is the Unruh Effect? Technically, it is one of the most interesting and controversial results of quantum field theory in a flat spacetime. It hypothesizes that a quantum field has the properties of a thermal state for an accelerated (i.e., not inertial or static) observer, and was discovered by William Unruh in 1976. So let's try to find a way to deal with this concept. I am going to do this with you, because by writing for you, I will be able to understand it better myself. First, let's review the four principles of classical thermodynamics, because we're going to need them:
0. If body "A" is in thermal equilibrium with body "B", and body "B" is in thermal equilibrium with body "C", then "A" and "C" are also in thermal equilibrium with each other. That is, neighboring systems interact within a thermodynamic model.
1. Energy can change form, but it cannot be created or destroyed (principle of conservation of energy).
2. Heat cannot spontaneously move from a cold body to a hot body.
3. The temperature of absolute zero can never be reached (In practice, no gas can ever reach the state of zero volume).
The four laws of black hole thermodynamics
A very precise analogy between classical thermodynamics and the laws governing black hole dynamics in general relativity was identified in the early 1970s. In particular, physicists came up with the four laws of black hole thermodynamics. They substituted black hole mass for energy, event horizon surface gravity for temperature, and event horizon area for entropy. It seemed like a stretch to say that black holes had thermal properties, but in 1975 Stephen Hawking theorized the emission of a thermal spectrum of quantum particles that is characterized by a temperature that is exactly proportional to the surface gravity of the event horizon: the Hawking radiation.
Hawking radiation
Let us try to explain Hawking radiation without the use of mathematical representations and without the use of the branches of theory. Imagine a thermal radiation produced by virtual particles that become real because of the interaction between the gravitational force of the black hole and its quantum field (always remember that a quantum state is a mathematical entity that provides a probability distribution for the results of any possible measurement on a system; we are talking about information and probabilities of obtaining certain results by making a measurement. Electrons in a quantum system do not have a position, but only the probability of a position). More specifically, the result of this interaction is the creation of particle-antiparticle pairs near the event horizon of the black hole. One particle of the pair enters the black hole. The other is returned to the quantum vacuum (the large region of space where the density of matter is very low, but not zero). The particle on its way out of the black hole is a drain on the black hole's energy, and so the black hole evaporates. And this is where the black hole information paradox comes in. According to Hawking's theoretical formulation, some of the information would be lost in the evaporation. But we will not explain the solution of the paradox here.
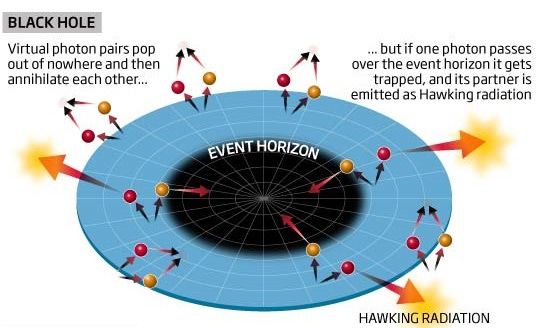
The hyperspace jumps
We have said that the Unruh effect theorizes that an observer in accelerated motion through the vacuum will actually encounter photons escaping from the vacuum. In QM, the observer (the measurement) conditions the state of the quantum system. The Unruh effect tells us that the energy required to transform virtual photons (virtual particles) into real photons (real particles) would be provided by the same accelerating observer. The observer would see the vacuum as a thermal bath of photons whose temperature would be proportional to the observer's acceleration (here we are with the properties of a thermal state). Remember the hyperspace jumps in Star Wars? The acceleration of the Millennium Falcon turned stars into streaks of light, well, replace stars with particles jumping out of nowhere and you have the Unruh Effect related to Hawking radiation. The question this theory tries to answer is whether quantum measurements change when the observer is in motion and the Unruh effect says "Yes, they change".
The link between General Relativity and QM?
If so, could this mysterious radiation produced by the acceleration be faster than the speed of light? Or could it be the link between General Relativity and QM? The Unruh Effect could only occur at speeds that are impossible for us to reach. So far, we have not been able to measure it and thus verify the theory. But if it is true that researchers at the University of Waterloo in Canada and the Massachusetts Institute of Technology (MIT) have found a way to do it at speeds that are within our grasp, there may be a change.
Ready for hyperspace? I can't wait!
Please let me know if you have more information on this topic! It'd be great to pass it on.
Super interesting stuff, Michael.
Some of these topics/theories are so complex but you lay them out in a way that makes them more accessible.
Thanks :)
Back in Uni, I had a friend of mine who transitioned from quantum to hyperspace physics. She and I had long talks, tiny bits of which I was allowed to use in my books.